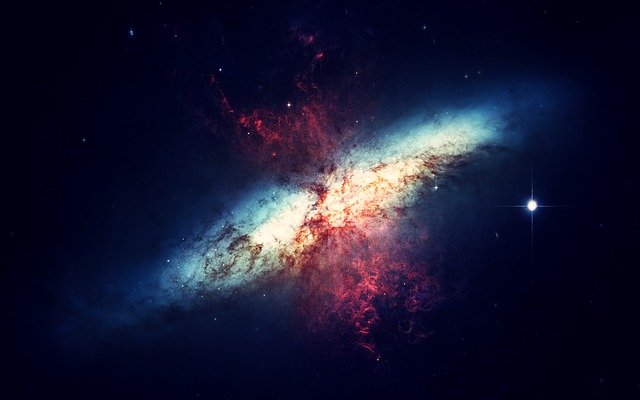
What is spacetime?
In physics, space-time is any mathematical model that combines the size of a space with a magnitude of one times four times the size. The space fabric is a concept model that combines the size of the spaces with the size of the fourth space. Space-time diagrams can be used to visualize related results, such as why different viewers see differently where and when events take place.
Until the 20th century, it was thought that the three-dimensional geometry of the universe (its geographical expression according to links, distances, and directions) was independent at the same time. The famous philosopher Albert Einstein helped to develop the concept of space-time as part of his theory of relativity. Before his pioneering work, scientists had two very different ideas about materialism: Isaac Newton's laws of physics described the movement of large objects, and James Clerk Maxwell's electric models described light structures. However, in 1905, Albert Einstein backed up the work with special correspondence in two letters:
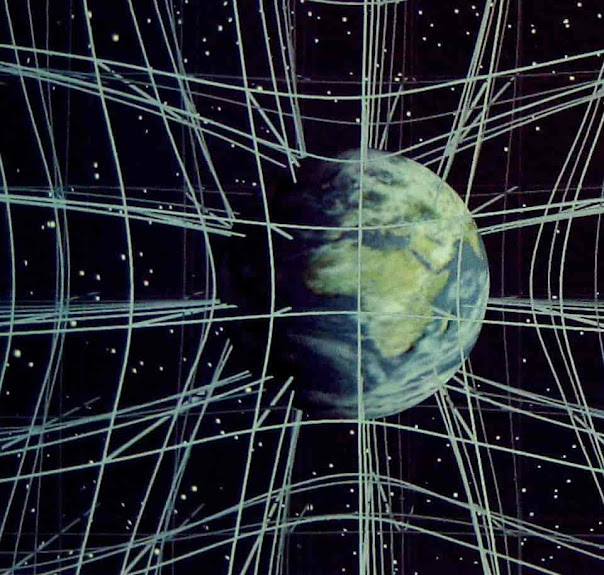
The laws of physics do not change (e.g., are the same) for all inactive systems (e.g., slow-moving frames)
The speed of light in space is the same for all viewers, regardless of the movement of the light source.
The logical consequence of uniting these postulates together is an inseparable combination of magnitude that has so far been thought to be independent - from time to time. Many contradictory results emerge: in addition to the independent movement of the light source, the speed of light remains constant regardless of the indicator frame on which it is measured; the distances and even the temporary order of the pairs of events change when measured in different independent states (this is one-time compliance), and the addition of line velocities is no longer successful.
Einstein developed his theory about kinematics (the study of moving bodies). His theory was the development of Lorentz's 1904 theory of electromagnetic and Poincaré's electrodynamic theory. Although these theories included estimates similar to those presented by Einstein (i.e., the Lorentz revolution), it was actually the types of ads that were suggested to explain the results of various tests - including the famous interferometer study Michelson - Morley - that were more difficult to get into existing paradigms.
In 1908, Hermann Minkowski - a former professor of mathematics at Einstein's youth in Zürich - introduced the geometric definition of a special relationship that affects time and the size of space into a fourth-dimensional space now called the Minkowski space. A key element of this definition is the official definition of space interval. Although distance and time intervals between events vary according to estimates made for different reference frames, the duration of the independent time frame is not independent of the reference framework not included in the record.
Minkowski's geometric definition of relativity would prove to be crucial to Einstein's growth in his 1915 overview of relativity, in which he showed how the magnitude and power of energy transformed a rare period of time into a myriad of Riemannian myths.
Definitions of spacetime
Classical non-relativistic mechanics treat time as the majority of the same universal volume everywhere, and different in space. The old mechanic assumes that time has a constant range of motion, independent of the movement of the viewer, or anything outside. Also, it assumes that space is Euclidean; assumes that space follows the geometry of common sense.
In the context of a special relationship, time cannot be separated by the size of the space, because the perceived rate at which an object passes over an object depends on the blocking of the object relative to the viewer. The general correlation also provides an explanation of how the gravitational fields can reduce the movement of an object as seen by an observer outside the field.
In the normal range, a position is defined by three numbers, known as magnitude. In the Cartesian link system, this is called x, y, and z. Position during space is called an event and requires the identification of four numbers: a three-dimensional space in space, and a space in time. The event is represented by a set of links x, y, z, and t. Space-time, therefore, has four elements. Mathematical events are zero lengths and represent one point at a time.
The particle path during space can be considered as a sequence of events. A series of events can be linked together to form a line that represents the continuation of a particle during space. That line is called the line of particles of earth.
Statistically, space-time is multiplied, that is, from a “flat” surface near each point in the same way, on a scale small enough, the globe appears flat. The largest scale, c (the so-called speed-of-light) refers to the distances measured in space by the distances measured in time. The magnitude of this scale (about 300,000 kilometers or 190,000 miles in space equal to one second at a time), and the fact that the time period is large, means that at normal speeds, not consistent with normal distances, few people can see much differently than they would if the Earth were Euclidean. It was because of the advent of critical scientific standards in the mid-1800s, such as the Fizeau study and the Michelson-Morley study, those bizarre contradictions began to emerge between observations compared to predictions based on speculative Euclidean space speculation.
By special relation, the viewer, in most cases, refers to a reference frame on which a set of objects or events are measured. This usage differs greatly from the standard English meaning of the word. Trusted frames are built in a non-local setting, and with the use of this term, it makes no sense to refer to the viewer as having a place. In Fig. 1‑1, imagine that the reference frame has a narrow window clock, synchronized within this reference frame, which extends indefinitely across all three dimensions of space. Any specific location within the rent is irrelevant. The latticework of clocks is used to determine the time and place of an event that takes place within the entire framework. The term watchman refers to a whole group of clocks associated with a single reference frame: 17–22 In this ideal case, each point in the space has a corresponding clock, and thus the clocks register each event immediately, without any time delay between the event and its recording. The real viewer, however, will notice a delay between signal output and its detection due to the speed of light. Sync clocks, in data reduction following the test, the time when the signal is received will be adjusted to indicate its own time if it was recorded with the correct clock.
In many books on special relationships, especially the old ones, the word "observer" is used in the general sense of the word. It is usually clear in the context that the meaning is used.
Physicists distinguish between what a person is experimenting with or seeing (after a person has released a signal delay), compared to what one can see with the naked eye without this correction. Failure to understand the difference between what a person is trying/seeing compared to what he or she sees is a source of great error among first-time relationship students
History of spacetime
In the mid-1800s, various experiments such as the location of Arago and different dynamics of light in the air concerning water were considered to prove the state of light in opposition to corpuscular theory. The distribution of waves is then considered to require the presence of a hand lifting device; concerning light waves, this was considered an aether of imaginary light. However, various attempts to locate the structures of this center of thought have produced conflicting results. For example, Fizeau's 1851 experiments showed that the speed of light in flowing water was less than the amount of light in the air and the speed of water in terms of the water index. Among other issues, the partial gravity of this experiment in refraction index (depending on the wavelength) has led to the unfortunate conclusion that the aether flows simultaneously at different speeds of different light colors. Michelson - Morley's famous experiment of 1887 did not show the influence of the Earth's motion variations by using the aether to guess at the speed of light, and the most probable explanation, the complete drag of the aether, contradicted the stellar view distortion.
George Francis FitzGerald in 1889, and Hendrik Lorentz in 1892, independently proposed that the visible bodies moving in a constant aether be physically affected by their passing, making an agreement in terms of the amount that was exactly what was needed to explain the negative results of Michelson-Morley's experiments. (No length changes occur in directions to moving directions.)
By 1904, Lorentz had developed the view that he came across mathematical terms similar to what Einstein would later find (meaning Lorentz's translation), but with a completely different translation. As a view of the power of flexibility (studying the power of torches and their effect on motion), his theory envisioned a physical paralysis of the story. : 163-174 Lorentz's statistics predicted a number he would call local time, with which he could explain light intensity, Fizeau experiments, and more. However, Lorentz views local time as a useful mathematical tool, such as a strategy, to facilitate the transition from one system to another.
Some physicists and mathematicians at the end of the century came close to reaching what is now known as space. Einstein himself noted, however, that as more and more people unravel the mysteries of the puzzle, "a special notion of relativism as we look back on its development in retrospect, was ready to be discovered in 1905.
An important example is Henri Poincaré,: 73-80,93-95 who in 1898 said that the union of two things is a matter of assembly. In 1900, he observed that Lorentz's "Local Time" was actually illustrated by moving clocks using a clear description of the function of clock synchronization that takes the speed of continuous light. In 1900 and 1904, he raised the issue of the authenticity of the aether by emphasizing the legitimacy of the object and called it the goal of relationships, and in 1905/1906 he developed mathematical proportions to fulfill Lorentz's idea of electrification. While discussing various theories about Lorentz's constant gravitational pull, he introduced a new concept of four-dimensional space-time by describing four different vectors, namely four positions, velocity, and four forces. He did not follow the 4-dimensional order in the following papers, but, said that the study appeared to "involve a great deal of pain of limited benefit", ultimately concluding that "three-dimensional language appears to be better suited to describing our world". Moreover, even after 1909, Poincaré continued to believe in the powerful interpretation of Lorentz's writings.: 163-174 For these and other reasons, many scientific historians say that Poincaré did not establish what is now called a special relationship.
In 1905, Einstein introduced a special relationship (with or without the use of space formalization techniques) in its modern understanding of space theory and time. While his results are statistically similar to those of Lorentz and Poincaré, Einstein has shown that Lorentz's transformation is not the result of interaction between the subject and the aether, but rather about the nature of space and time itself. He obtained all his results by seeing that all doctrine can be built into two books: The basis of relation and the goal of the intensity of light speed.
Einstein made his analysis of kinematics (the study of moving bodies without reference to energy) more powerful. His work on the subject was filled with vivid illustrations involving the exchange of light signals between moving clocks, careful measurements of the length of moving rods, and other such examples.
Also, Einstein in 1905 replaced previous attempts at power relations by introducing a general balance of weight and power, which contributed to his subsequent formulation of the equality principle in 1907, declaring equality of non-existent magnitude and gravity. By using a balance of power, Einstein demonstrated, moreover, that gravity is equal to the content of its power, which was one of the first effects on building normal relationships. While it may appear that at first, he did not consider geometry about space-time,: 219 in the continuation of the normal relationship Einstein completely incorporated space order.
When Einstein published in 1905, one of his competitors, his former professor of mathematics Hermann Minkowski, had also come to the forefront of a very special relationship. Max Born recounted a meeting he had with Minkowski, who wanted to be Minkowski's student/participant:
Minkowski was concerned about the state of electrodynamics after Michelson's disruptive research at least since the summer of 1905, when Minkowski and David Hilbert led a high-level conference attended by prominent scientists at the time to study Lorentz's papers, Poincaré et al. However, it is not clear at all when Minkowski began the geometric formation of the special relationship that would be named after him, or how much he was influenced by the magnitude of Poincaré's four-dimensional interpretation of Lorentz's revolution. And it is unclear whether he ever appreciated Einstein's critical contribution to understanding the changes being made in Lorentz, thinking of Einstein's work as an extension of Lorentz's work.
The concept of local time with the Lorentz group is closely linked to certain types of scenes, hyperbolic, or similar geometry, and their conversion groups already developed in the 19th century when constant periods such as rest periods were used. On 5 November 1907 (more than a year before his death), Minkowski introduced his geometric definition of space-time in a speech to the Göttingen Mathematical Society entitled, The Relativity Principle (Das Relativitätsprinzip). On September 21, 1908, Minkowski delivered his famous speech, Space and Time (Raum und Zeit), to the German Society of Scientists and Physicians. The opening words of Space and Time include Minkowski's famous statement that "From now on, space itself, and its time, will be completely reduced to a mere shadow, and only a certain kind of unity of the two will preserve freedom." Space and time included the first public presentation of local time diagrams, as well as a striking indication that the concept of constant motion, as well as the powerful view that light speed is exhausted, allows for a unique special connection.
Einstein, meanwhile, was initially opposed to Minkowski's translation in a special relationship, being regarded as überflüssige Gelehrsamkeit (unnecessary reading). However, to complete his search for a common relationship that began in 1907, the geometric interpretation of the relationship proved to be important, and in 1916, Einstein fully acknowledged his debt to Minkowski, whose explanations greatly helped to transition to normal relations. : 151-152 As with other types of space-time, such as the fixed time interval for normal relationships, the interval space for special relationships today is known as Minkowski space.
ATOMS OF SPACETIME
Heating random movements of very small particles, such as gas molecules. Because black holes can heat and cool, it makes sense that they have parts - or, more importantly, a thin structure. And because the black hole is an empty space (according to a common relationship, an infinite object passes through the horizon but cannot last long), the parts of the black hole must be parts of the space itself. Obviously, as the atmosphere of the empty space can look, it has a great deal of difficulty in hiding.
Even ideologies that are supposed to save the general idea of local time end up concluding that there is something hidden behind an empty element. For example, in the late 1970s, Steven Weinberg, now at the University of Texas at Austin, sought to define gravity in the same way as other natural forces. You still find that space-time is completely changed on its best scales.
Scientists first discovered tiny space as a spacecraft. They thought that if you approached the scale of Planck, the unimaginable size of about 10-35 meters, you would see something like a chessboard. But that would not be fair. Among other things, the grid lines of the chessboard space may provide certain indications above others, creating asymmetries that contradict the special view of the relationship. For example, different colors of light can travel at different speeds - such as on a glass plate, which illuminates light with its own colors. While the results on the small scales are often difficult to detect, the violation of the relationship will actually be obvious.
The thermodynamics of the dark holes remove even more doubt from the local image as a simple drawing. By measuring the operating temperature of the system, you can calculate its components, at least legally. Lose energy and look at the thermometer. When a temperature rises, that energy must be dispersed in relatively small molecules. In fact, he measures the entropy of the system, which represents its minimum weight.
If you pass this standard test, the number of molecules increases with the volume of the object. That's the way it is: If you increase the size of a sea ball by 10 degrees, you will have a thousand-fold molecule inside it. But if you increase the radius of a black hole by 10 degrees, the target number of molecules increases by only 100. The number of "molecules" made should not be equal to its volume but to its surface. The black hole may look three-dimensional, but in reality, it behaves as if it looks double.
This strange effect goes under the holographic word because it reminds us of the hologram, which presents itself to us as a three-dimensional object. On closer inspection, however, it turns out that it is an image produced by a double-sided sheet. If the holographic system counts small celestial elements and their contents - as a general scientist, though not universally accepted, - it should take more to build space than to reassemble its fragments.
Complete partial relationships are rarely straightforward, however. The H2O molecule is not just a small fraction of water. Think of what a liquid does: it flows, forms droplets, carries waves and waves, and then freezes and boils. Each H2O molecule cannot do that: those are collective behaviors. Similarly, space building blocks do not need to have space. "Atoms in space are not the smallest parts of the universe," said Daniel Oriti of the Ludwig Maximilian University of Munich in Germany. “They are the builders of space. The geometric features of space are new, integrated, probably the elements of a system made up of many such atoms. ”
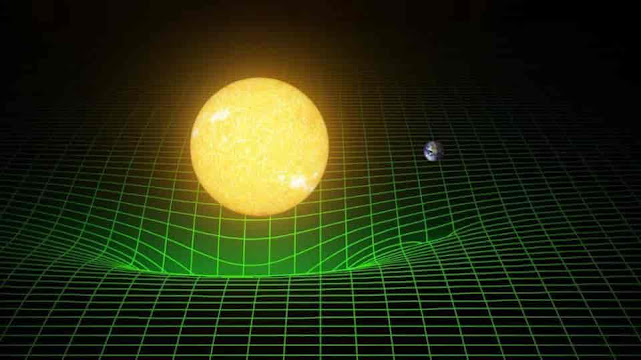
What exactly those building blocks are based on this idea. In loop quantum gravity, they are volume quanta combined through quantum terms. In the sense of ropes, they are fields corresponding to those of the more permanent electromagnetism followed by a leading thread or power loop - a namesake string. In M-theory, which is related to rope theory and can be supported, they are a special kind of particle: the membrane is shriveled to a certain degree. According to the theory laid down, it is the web-related events of cause and effect. In the sense of amplituhedron and other means, there are no building blocks at all - at least not in the ordinary sense.
Although the planning principles of these theories differ, they all strive to support the so-called relationalism of 17th- and 18th-century German philosopher Gottfried Leibniz. In broad terms, commercial relationships assume that space arises from a particular pattern of interactions between objects. In this view, space is a jigsaw puzzle. You start with a large pile of pieces, see how they connect and place them appropriately. If the two pieces have the same features, the same color, they may be closer; if they differ strongly, you set them apart differently. Naturalists often portray this relationship as a network with a specific connection pattern. Relationships are determined by quantum theory or other principles, followed by spatial planning.
Category switching is one of the standard themes. If space is compacted, it may be subdivided, too; then its building blocks can be organized into something that doesn’t look like space. “Just as you have different classes of matter, such as ice, water, and water vapor, atmospheric atoms can also be classified into different categories,” says Five Padmanabhan of the Inter-University Center for Astronomy and Astrophysics in India. In this view, black holes can be areas where space melts. Well-known ideas are declining, but a common view can explain what is happening in a new phase. Even when space reaches its end, physics continues.
DOWN THE BLACK HOLE
The magnetic field of the kitchen reflects the problem that scientists are facing. It can hold a piece of paper against the gravitational force of the Earth. Gravity is weaker than magnetic fields or electric or nuclear power. Any quantum effects it has are still weak. The only concrete evidence that these processes occur at all is a very original dot pattern - which is thought to be partly due to fluctuations in the force of gravity.
Black holes are an excellent test condition for quantum gravity. "It's the closest thing we have to do in an exam," said Ted Jacobson of the University of Maryland, College Park. He and other theorists study black holes as doctrinal fulcrums. What happens if you take equations that work well under laboratory conditions and put them in the worst-case scenario? Will some hidden error occur?
A common connection predicts that an object falling into a black hole is pressed indefinitely as it approaches the center - a static cul-de-sac called unity. Theorists cannot emphasize the course of an object more than once; its timeline ends there. Even the word “exist” is problematic because the very term that describes the place of unity is no more. Researchers hope that quantum theory can focus on the microscope at that point and track what happens to it.
Outside of the pit boundary, the matter is less pressured, gravity is weaker and, by all rights, well-known laws of physics must still hold. Therefore, it is very confusing that they do not. The black hole is designed with the limit of the event, the point of no return: the issue that falls inside can never come out again. The descent cannot be undone. That is a problem because all known laws of basic physics, including quantum mechanics as commonly understood, are reversed. At least legally, you should be able to reverse the movement of all the particles and get what you have.
The same conundrum was encountered by physicists in the late 1800s when they thought of “black body” figures, which are neatly arranged like a hole filled with electromagnetic radiation. James Clerk Maxwell's theory of electromagnetism predicted that such a thing could absorb all the radiation involved in it and that it would never mix with the elements around it. "It could take a constant heat from a well stored at a constant temperature," explains Rafael Sorkin of the Perimeter Institute for Theoretical Physics in Ontario. In hot terms, it would have a completely zero temperature. This conclusion contradicts the perception of real black bodies (like an oven). Following the work done by Max Planck, Einstein has shown that the black body can achieve thermal equilibrium if the radiation energy comes in different units or quanta.
Physicists have been trying for nearly half a century to achieve a uniform solution of black holes. The late Stephen Hawking of the University of Cambridge took a big step in the mid-1970s when he incorporated quantum theory into the field of radiation around black holes and showed that they had nausea temperature. As a result, they can not only absorb but also release energy. Although his analysis revealed black holes inside the thermodynamics compound, it exacerbated the problem of instability. The radiation emanating comes from just outside the hole and does not contain information about the inside. It is a random heatwave. If you reverse the process and put the power inside, the things that fell will not come out; you will just get more heat. And you can't imagine that real things still exist, they just get stuck in the hole, because as the hole emits rays, it shrinks and, according to Hawking's analysis, eventually disappears.
This problem is called the information puzzle because the black hole destroys information about non-disruptive particles that can allow you to reverse their movement. If black hole physics is reversed, something has to be restored, and our sense of local time may need to change to allow for that.
THE WEBS HAVE BEEN CONVERTED
A major fulfillment of recent years — and the one that has gone beyond the basics of disciplinary action — is that the right relationship involves quantum entanglement. The type of overhead connection, which is internal to the quantum machine, the grip seems to be much older than space. For example, an experimentalist can create two particles that fly in opposite directions. When they are busy, they stay organized no matter how far away they are.
Traditionally when people talk about the gravitational force of quantum, they were referring to the appearance of quantum, quantum flexibility, and almost all other quantum effects in the book - but never to be trapped in quantum. That changed when black holes forced the problem. Throughout the life of the black hole, trapped particles fall in, but after the hole is fully evaporated, their outside partners are left confused - nothing. "Hawking should have called it a tangible problem," said Samir Mathur of Ohio State University.
Even in an empty area, with no particles around the area, electricity and other sectors are trapped inside. If you rate the field in two different places, your reading will shake in a random but consistent way. And if you divide a circuit into two parts, the pieces will be joined, and the level of interaction depends on the size of the geometry they only have in common: the location of their visible connector. In 1995 Jacobson argued that constellation provides a link between the existence of matter and geometry of space - that is, it may explain the law of gravity. "Too much catch means weak gravity - that is, a hard time in space," he says.
Many forms of quantum gravity - above all, the theory of the series - now see censorship as a priority. The rope concept uses the holographic principle not only in dark holes but also in the universe, providing a recipe for how to create space - or at least one of them. For example, a two-dimensional space can be fitted with fields that, when properly constructed, increase the size of the space. The first two-dimensional space will serve as the boundary of a broad state, known as bulk space. And clutter is what combines a lot of space into something tangible.
In 2009 Mark Van Raamsdonk of the University of British Columbia gave a good debate on this process. Suppose border fields are not bound - they form unrelated systems. They are associated with two different universities, with no way to move between them. When these systems get stuck, it appears that a tunnel, or caterpillar, opens between these areas, and the spacecraft can move from one place to another. As the catch rate increases, the caterpillar decreases in length, pulling the whole area together until you no longer talk about it as two universities. "The emergence of a vast space in space is directly related to the holding of these liberal education degrees," Van Raamsdonk said. When we see the interconnectedness of electricity in other spheres, it is the remnants of a bond that binds space together.
Many other aspects of space, in addition to its position, can also indicate clutter. Van Raamsdonk and Brian Swingle, now at the University of Maryland, argue that tangible globalization means a global gravitational pull - that it affects everything and cannot be explored. About black holes, Leonard Susskind of Stanford University and Juan Maldacena of the Institute for Advanced Study in Princeton, N.J., suggested that the interaction between the black hole and the radiation emitted formed a caterpillar - the back door to the hole. That can help keep track of details and ensure that black hole physics is reversed.
While these concepts of a series of strings only apply to certain geometries and form only the size of a single space, some researchers have tried to explain how the whole space can emerge at first. For example, ChunJun Cao of the University of Maryland and Spyridon Michalakis and Sean M. Carroll, both at the California Institute of Technology, began with a very small description of the program, which was done without direct reference to time or even anything. If it has the right assembly pattern, the system can be broken down into sections that can be identified as different time zones. In this model, the entry-level defines the concept of a local distance.
In physics and, in general, in the natural sciences, space and time are the basis of all ideas. However, we have never seen the exact space-time. Instead, we take its presence from our daily experiences. We think the most expensive account of the situations we see is the one that works in space. But the key lesson of quantum gravity is that not all objects fit perfectly in space. Physicists will need to find a new structure, and when they do, they will have completed the revolution that began more than a century ago with Einstein.
2 Comments
hey
ReplyDeletenice content
Thanks bro!
DeleteThanks for your feedback.